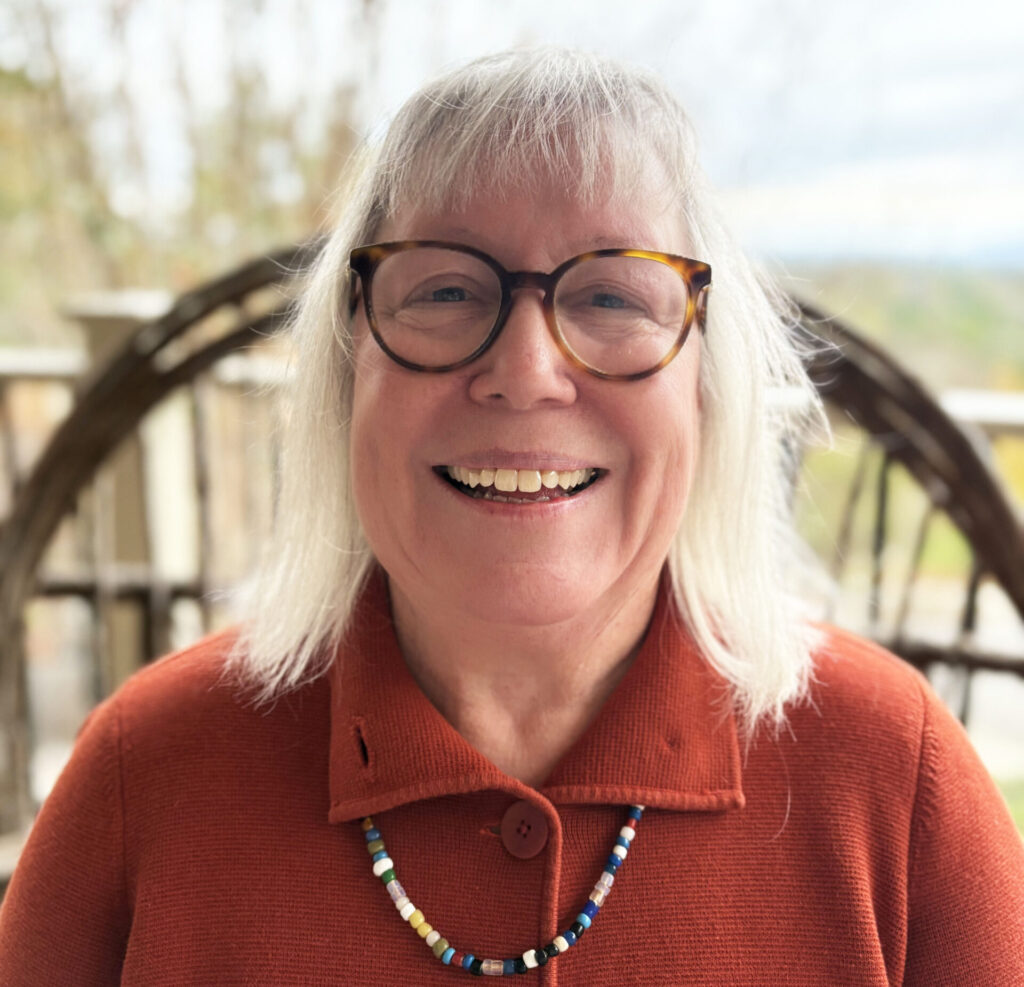
November is National Native American Heritage Month. In this post, we spotlight the exceptional career of one of our Native American community members: Robbie Hood.
Hood is an atmospheric scientist with over 30 years of experience at the National Aeronautics and Space Administration and the National Oceanic and Atmospheric Administration. She is a member of the Cherokee Nation of Oklahoma.
Can you tell us a few highlights of your current or most recent work?
I am a Cherokee meteorologist who worked with NASA and NOAA for a combined total of 30 years. Now in retirement, I am collaborating with NCAR personnel to explore how low-cost 3-D printed weather stations could be used by Indigenous communities for student training and tribal decision-making. I have also been collaborating with the Rising Voices Center for Indigenous and Earth Sciences to plan the program for the 2025 AMS Heather Lazrus Symposium to be held during the 105th AMS Annual Meeting in New Orleans. In this symposium, I will be moderating a panel discussion focused on cultivating tribal and community partnerships within the weather, water, and climate enterprise.
What was an important moment in your early career?
I started my meteorological career as scientific programmer, but I was given an opportunity to step out of my comfort zone at NASA. I became the project scientist for a new passive microwave aircraft instrument that could observe precipitation within thunderstorms and hurricanes. This move gave me the opportunity to work with and, eventually, lead teams of renowned scientists, engineers, and pilots during large international weather field experiments that were conducted in places like Australia, Brazil, Costa Rica, Cape Verde, and the Marshall Islands. Along the way, I got to fly through four different hurricanes, all because I initially stepped out of my comfort zone.
What is something you’re proud of professionally?
My experience leading weather field experiments led me to become the manager of a NOAA program to test the potential benefit of drones and remotely piloted aircraft for operational application. In this role, I was able to organize and fund a multi-million-dollar field experiment to test, for the very first time, the capabilities of a high-altitude Global Hawk remotely piloted aircraft that was controlled from a central command center in Virginia to fly over and observe the characteristics of hurricanes and tropical storms in the Atlantic Ocean and Gulf of Mexico. During these missions, relevant data were successfully sent in near-real time to meteorologists at the National Hurricane Center to assist in their forecasts. Later research studying the impact of Global Hawk data assimilated into weather prediction models demonstrated positive results.
Are there ways in which your Native heritage has influenced or enriched your career?
I credit my Cherokee mother for teaching me to listen closely to how people tell their stories and pay attention to their feelings and expressions as they talk. She thought this would help me better understand who they are and how things looked from their point of view. These skills have helped me build good working relationships and strong collaborations throughout my career.